Evolution of manganese-based cathode chemistry in rechargeable batteries.
2021-06-25
The global climate change crisis combines the impacts of excessive use of fossil fuels with anticipated severe socioeconomic and political consequences1. Replacing carbon-based fossil fuels with clean energy harvested from renewable resources (solar, wind, tidal, geothermal and so on) is the only viable long-term solution to address climate change. Due to the intermittent nature of renewable energies, energy-storage solutions should be implemented alongside. Lithium-ion batteries (LIBs) are one of the most promising technologies.
In LIBs, the positive electrode (that is, the cathode) comprises the highest cost fraction of all cell components. Ironically, it is also the most problematic component from a technical point of view2. Replacing the less abundant transition-metal oxides used as the cathode in LIBs with more abundant analogues, such as manganese (Mn), is considered a holy grail for the development of the next-generation LIBs3. The rich chemistry that Mn oxides offer is not trouble-free though, as it shows some reactivity and unwanted side-reactions responsible for capacity loss and cell failure. Writing in Nature Sustainability, Zhu et al.4 propose an innovative approach to control the extent of unwanted side-reactions of Mn-based oxide cathode materials for use in LIBs, and so mitigating one of the key issues in the implementation of Mn-based cathode chemistry to large-scale battery production.
The first generation of LIBs used a lithium-cobalt oxide cathode and a graphite anode5. However, due to the high cost, limited terrestrial distribution of cobalt deposits, and high-toxicity of cobalt compounds, experts worked on a partial replacement of cobalt with nickel and manganese in the design of subsequent generations of LIBs6. Still, cathode chemistries comprising nickel compounds are not entirely free from the complications relevant to cobalt analogues, further downgraded with inferior cyclability due to the higher reactivity of nickel. Among the transition metals, manganese is an attractive choice for large-scale battery fabrication considering its abundance and lower toxicity levels.
From a chemical point of view, manganese oxide chemistry is vibrant due to the availability of various oxidation states and diversity in chemical bonding within the Mn–O framework. The common oxidation states of Mn deemed suitable for battery applications are 3+ and 4+. For electronic structure reasons, the Mn cation in the 3+ state is less symmetric than the 4+ state due to the Jahn–Teller effect, which refers to the distortion of molecules from a geometry of high symmetry to a less symmetric one (Fig. 1a). As a result, the transition of Mn species between the 3+ and 4+ states introduces substantial structural instability that is the source of particle cracking and dissolution of the cathode active material, leading to cell performance degradation and failure (Fig. 1b)7,8,9.
Fig. 1: Evolution of manganese-based cathode chemistry in rechargeable batteries.
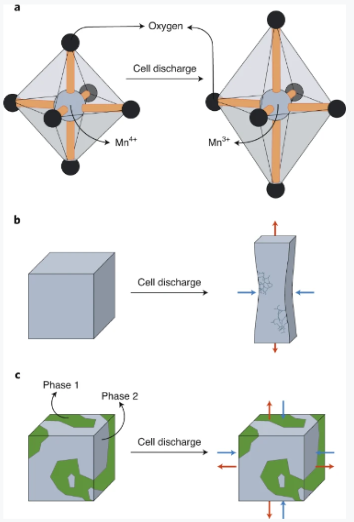
a, The reduction of Mn4+ to Mn3+ as a result of cell discharge leads to the emergence of Jahn–Teller distortion, reducing the symmetry of the octahedral MnO6 atomic fragment in Mn4+ to tetragonal in the Mn3+ analogue. b, Cathode crystallite cracking during the cell discharge as a result of crystal strain and Jahn–Teller distortion of manganese ions. c, The two manganese dioxide phases with a shared interface suppress the crystallite strain and subsequent cell failure. The red and blue arrows indicate, respectively, the tensile and compressive stresses induced during the cell discharge as a result of the Jahn–Teller distortion.
Full size image
The approach presented by Zhu et al. seeks to mitigate the complications associated with Mn3+ oxide through an innovative interface engineering approach that partially disturbs the orbital ordering leading to the Jahn–Teller distortion (Fig. 1c). The proposed method converts a parent spinel-type Mn3O4 phase to two LiMnO2 product phases (that is, polymorphs) via electrochemical cycling in a Li-ion cell. Interestingly, the crystal structure modification occurred at room temperature using the electrochemical driving force. The soft-chemical approach used in this work allows stabilizing interfaces that are responsible for the improved performance.
The two LiMnO2 polymorphs, when synthesized and used independently in LIBs, suffer from rapid capacity loss due to the reasons mentioned above. However, the cell performance is notably improved when the two phases form in situ from a common parent phase and share an interface between their crystal domains. According to their data, the mosaic nature of the active material particle with a shared interface between the two phases prevents the propagation of the atomic orbital ordering, leading to enhanced structural stability. As a result, the cathode design delivers a high capacity of 223 mAh g−1 at an average potential of 3.3 V and retains 88% of the initial capacity after 1,000 cycles. The performance level reported in this work surpasses those of individual LiMnO2 phases that hardly reach a few tens of cycles.
The new methodology proposed by Zhu et al. opens up an avenue for the implementation of Mn-based LIBs for high-energy and high-power density applications sought after by mobile and stationary energy storage markets, given the high abundance of manganese deposits, the availability of the required infrastructures for the processing of manganese-based cathodes, and the low toxicity of manganese compounds compared to cobalt and nickel counterparts. Despite the promising results presented, more work is left to make the most out of the reported findings. For example, it remains a task for quantum chemists and materials scientists to explain how the interface engineering can suppress the development of long-range Jahn–Teller distortion. Furthermore, new strategies are needed to adapt the proposed electrochemical synthesis for large-scale material production methods. It is expected that improvement in cell design that lowers the cost of the energy storage system and improves its sustainability will smoothen the transition from fossil-fuel-based energy technologies to cleaner alternatives at a faster pace.